Compressible Multiphase Flows
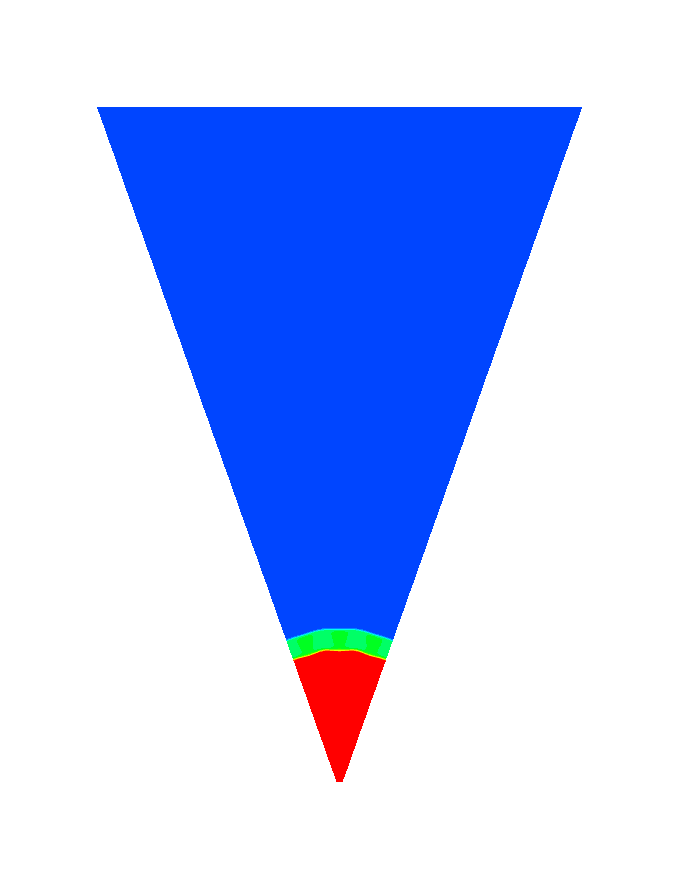
Nonlinear Rayleigh-Taylor Instability of a Cylindrical Interface in Explosion Flows
S. Annamalai, M. Parmar, Y. Ling, and S. Balachandar,
Journal of Fluids Engineering-Transactions of ASME 136, 060910 (2014)
Abstract: The nonlinear growth of instabilities of an outward propagating, but decelerating, cylindrical interface separated by fluids of different densities is investigated. Single mode perturbations are introduced around the contact-surface, and their evolution is studied by conducting inviscid 2D and 3D numerical simulations. In the past, a significant amount of work has been carried out to model the development of the perturbations in a planar context where the contact surface is stationary or in a spherical context where a pointsource blast wave is initiated at the origin. However, for the finite-source cylindrical blast-wave problem under consideration, there is a need for a framework which includes additional complexities such as compressibility, transition from linear to nonlinear stages of instability, finite thickness of the contact interface (CI), and time-dependent deceleration of the contact surface. Several theoretical potential flow models are presented. The model which is able to capture the above mentioned effects (causing deviation from the classical Rayleigh-Taylor Instability (RTI)) is identified as it compares reasonably well with the DNS results. Only for higher wavenumbers, the early development of secondary instabilities (Kelvin-Helmholtz) complicates the model prediction, especially in the estimation of the high-density fluid moving into low-density ambient.
A scaling analysis of added-mass and history forces and their coupling in dispersed multiphase flows
Y. Ling, M. Parmar, and S. Balachandar
International Journal of Multiphase Flow 57, 102-114 (2013)
Abstract: Accurate momentum coupling model is vital to simulation of dispersed multiphase flows. The overall force exerted on a particle is divided into four physically meaningful contributions, i.e., quasi-steady, stress-gradient, added-mass, and viscous-unsteady (history) forces. Time scale analysis on the turbulent multiphase flow and the viscous-unsteady kernel shows that the integral representation of the viscous-unsteady force is required except for a narrow range of particle size around the Kolmogorov length scale when particle-to-fluid density ratio is large. Conventionally, the particle-to-fluid density ratio is used to evaluate the relative importance of the unsteady forces (stress-gradient, added-mass, and history forces) in the momentum coupling. However, it is shown from our analysis that when particle-to-fluid density ratio is large, the importance of the unsteady forces depends on the particle-to-fluid length scale ratio and not on the density ratio. Provided the particle size is comparable to the smallest fluid length scale (i.e., Kolmogorov length scale for turbulence or shock thickness for shock-particle interaction) or larger, unsteady forces are important in evaluating the particle motion. Furthermore, the particle mass loading is often used to estimate the importance of the back effect of particles on the fluid. An improved estimate of backward coupling for each force contribution is established through a scaling argument. The back effects of stress-gradient and added-mass forces depend on particle volume fraction. For large particle-to-fluid density ratio, the importance of the quasi-steady force in backward coupling depends on the particle mass fraction; while that of the viscous-unsteady force is related to both particle mass and volume fractions.

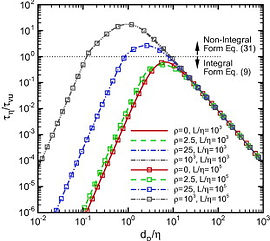
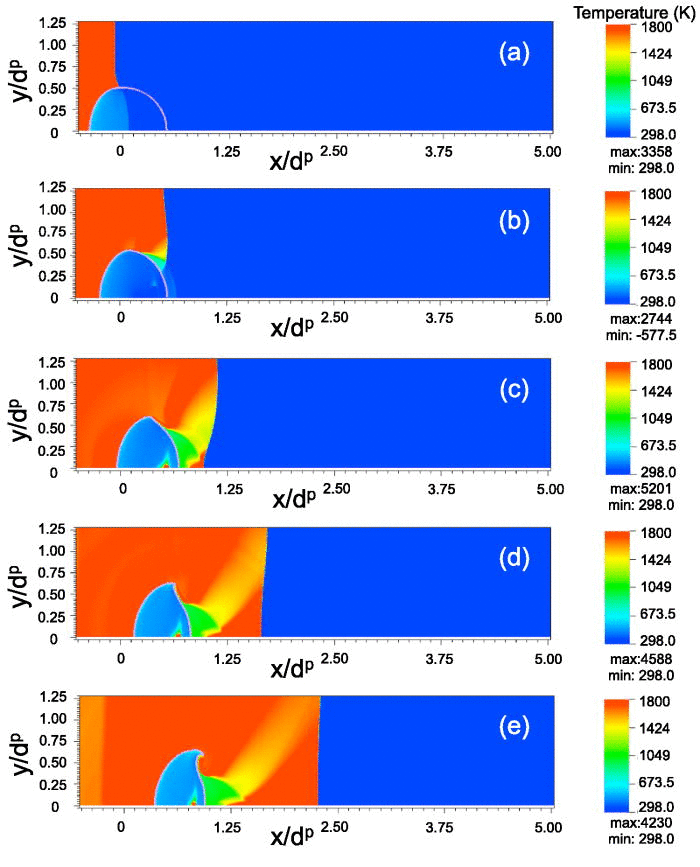
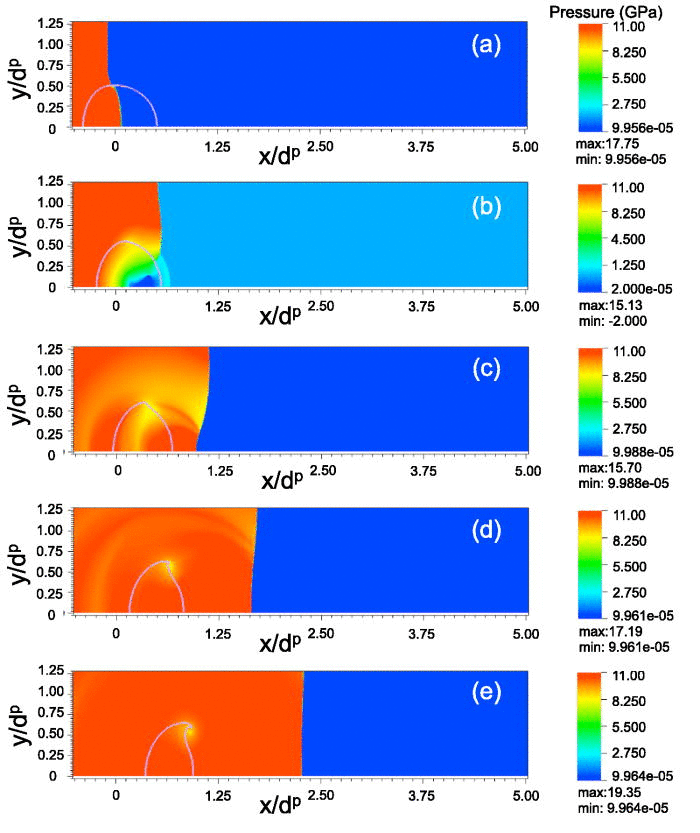
Shock interaction with a deformable particle: Direct numerical simulation and point-particle modeling
Y. Ling, A. Haselbacher, S. Balachandar, F. M. Najjar, and D. S. Stewart
Journal of Applied Physics 113, 013504 (2013)
Abstract: The interaction of shock waves with deformable particles is an important fundamental problem. In some applications, e.g., the detonation of explosives loaded with metal particles, the pressure behind the shock wave can be significantly larger than the yield strength of the particle material. This means that particles can deform severely during their interaction with the shock wave. The experimental and theoretical studies of shock interaction with deformable particles (SIDP) are extremely challenging because of its highly transient nature. As a result, no accurate model exists yet that can be used in simulations. The objective of this paper is to develop a simple point-particle model that accurately captures the unsteady force and heat-transfer in SIDP. In the development of this model, we build on earlier models by Ling et al. (Int. J. Multiphase Flow 37, 1026–1044 (2011)) for the unsteady force and heat-transfer contributions for rigid particles. Insights gained from direct numerical simulations (DNS) guide the extension of these models to deforming particles. Results obtained with the extended model for the interaction of a deforming particle with a shock wave and a Chapman-Jouguet detonation wave compare well with DNS results and therefore offer significant improvements over standard models.
Interaction of a planar shock wave with a dense particle curtain: Modeling and experiments
Y. Ling, J. L. Wagner, S. J. Beresh, S. P. Kearney, and S. Balachandar
Physics of Fluids 24, 113301 (2012)
Abstract: The interaction of a planar shock wave with a dense particle curtain is investigated through modeling and experiments. The physics in the interaction between a shock wave with a dense gas-particle mixture is markedly differently from that with a dilute mixture. Following the passage of the shock wave, the dense particle curtain expands rapidly as it propagates downstream and pressures equilibrate throughout the flow field. In the simulations, the particles are viewed as point-particles and are traced in a Lagrangian framework. A physics-based model is then developed to account for interphase coupling. Compared to the standard drag law, four major improvements are made in the present interphase coupling model to take into account: (1) unsteady force contributions to particle force; (2) effect of compressibility on hydrodynamic forces; (3) effect of particle volume fraction on hydrodynamic forces; (4) effect of inter-particle collision. The complex behavior of the dense particle curtain is due to the interplay between two-way coupling, finite particle inertia, and unsteady forces. Incorporation of these effects through significant modeling improvements is essential for the simulation results to agree well with the experimental data. As a result of the large pressure gradient inside the particle curtain, the unsteady forces remain significant for a long time compared to the quasi-steady force and greatly influence the particle curtain motion.

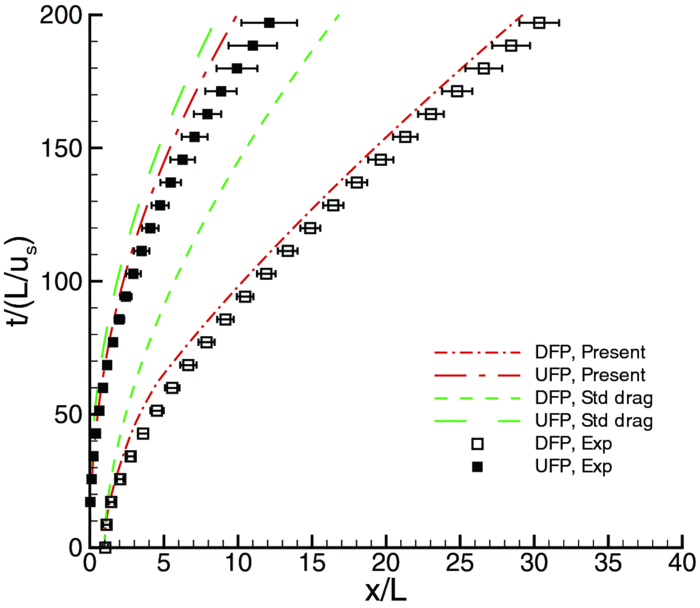
Importance of unsteady contributions to force and heating for particles in compressible flows. Part 2: Application to particle dispersal by blast waves
Y. Ling, A. Haselbacher, and S. Balachandar
International Journal of Multiphase Flow 37, 1013-1025 (2011)
Abstract: Particle dispersal by blast waves is an interesting phenomenon. A model problem, i.e., a sudden release of a compressed gas–particle mixture contained in a spherical container, is employed to investigate the fundamental physics of particle dispersal. The problem is simulated by the multiphase flow models proposed in Part 1 of this article that include unsteady contributions in momentum and energy exchange between gas and particles. At early times, when particles are accelerated in the expansion fan, unsteady force and heating contributions are much larger than the corresponding quasi-steady contributions. Consequently, neglecting unsteady contributions leads to significant errors in particle front location (the boundary of the particle cloud). The complex wave interactions in the flow have strong influence on the particle motion. As a result, the particle motion is a non-monotonic function of particle density or diameter and the evolution of particle concentration is non-uniform and unsteady.
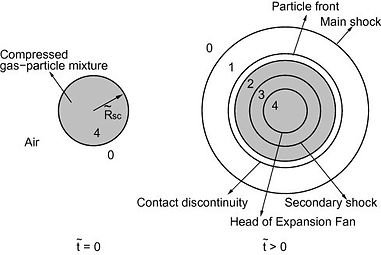
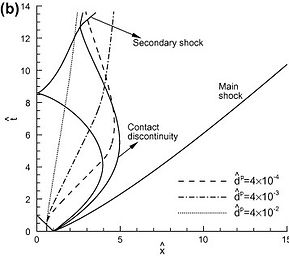
Importance of unsteady contributions to force and heating for particles in compressible flows. Part 1: Modeling and analysis for shock-particle interaction
Y. Ling, A. Haselbacher, and S. Balachandar
International Journal of Multiphase Flow 37, 1026-1044 (2011)
Abstract: Shock–particle interaction is an important phenomenon. The interaction can be accurately resolved by direct numerical simulations. However, as the length scales of interest are much larger than the particle size in many applications, fully resolving the flow around the particle is impractical. Therefore, rigorous model for momentum and energy exchange in the interaction is very important. Shock–particle interaction is strongly time-dependent, so unsteady mechanisms play important roles in momentum and energy transfer. A model that includes unsteady contributions to force and heating is proposed. The model is used to investigate particle interactions with a planar shock wave and a spherical shock wave. The peak values and the net effects of unsteady contributions are used to measure their importance. The results show the peak values of unsteady contributions are much larger than the quasi-steady ones for a wide range of particle parameters. The net effects of unsteady contributions are important when the particle-to-gas density ratio is small. For the flow behind the spherical shock is unsteady and non-uniform, unsteady contributions have long-time influence on the particle evolution.
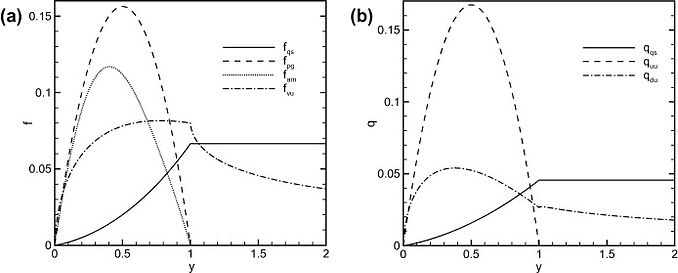
A numerical source of small-scale number-density fluctuations in Eulerian-Lagrangian simulations of multiphase flows
Y. Ling, A. Haselbacher, and S. Balachandar
Journal of Computational Physics 229,1828-1851 (2010)
Abstract: Eulerian–Lagrangian simulations of multiphase flow are known to suffer from two errors that can introduce small-scale fluctuations in the number-density of the dispersed phase. These errors can be reduced by increasing the number of particles in the simulation. Here, we present results to demonstrate that a third error exists that can also generate small-scale number-density fluctuations. In contrast to the two known errors, this error cannot be lowered by increasing the number of particles. Analysis shows that this error is caused by spatial variation at the subgrid scale in the interpolation error of the fluid velocity to the particle location. If the particle velocity divergence is zero, the particle concentration error remains at the subgrid scale. However, if particles preferentially accumulate either due to their inertia or due to divergence of the underlying fluid-velocity field, this error manifests as number-density fluctuations on the grid scale. The only mechanism of reducing these errors is through higher-order accurate interpolation. By studying two model problems, estimates for the errors are derived. These estimates are shown to be quite accurate for simulations of shock and expansion waves interacting with particles.
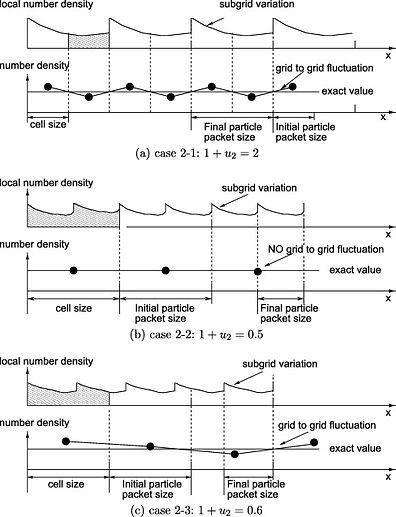